Abstract
It was previously believed that exosomes, membrane-bound extracellular vesicles released by cells, were a waste disposal mechanism resulting from cell damage or from by-products of cell homeostasis. However, in recent years, studies have revealed that exosomes play a key role in cell-to-cell communication, cell homeostasis and tumor progression and inhibition. Exosomes contain complex cargos of protein, lipids and nucleic acids which they are able to deliver to specific target cells to alter their function and physiology [1]. This newfound role of exosomes makes them interesting candidates for drug delivery systems where they can be engineered and loaded with a variety of cargos to deliver drugs to specific cell types. Additionally, unmodified exosomes derived from certain cells are thought to have innate therapeutic potential due to their unique compositions. This review focuses on the potential use of exosomes as cancer therapeutics and the challenges this entails.
1) Introduction
Most eukaryotic cells release membrane-bound vesicles known as exosomes which are involved in a wide variety of biological processes. These 40-160 nm vesicles are enclosed within a single outer membrane and can carry a variety of cargos such as lipids, metabolites, proteins, and nucleic acids. There is significant heterogeneity between different exosomes and their compositions and it is believed that their origin will ultimately determine their biological functions [2]. In the past few years, interest in the exosome field has grown rapidly, with exosomes implicated in several important biological processes. Firstly, exosomes can act as a signaling pathway by stimulating cells with ligands in their outer lipid layer as well as modify a cell’s phenotype by transferring membrane receptors between cells. Secondly, exosomes can exchange mRNA, miRNA, proteins, and even small organelles between cells. This has sparked interest in their use as therapeutic “Ubers” wherein they can be engineered to carry drugs, bioactive compounds, or genetic material to target cells.
1.1) Exosomes: biogenesis and composition
Exosomes are formed when the late endosomal membrane retracts and creates intraluminal vesicles (ILVs) within the endosome, also known as a multivesicular body (MVB). During this membrane budding process, certain proteins are incorporated on the membrane and other cytosolic payloads are engulfed and enclosed to create the ILVs. When the MVB fuses with the plasma membrane, these vesicles are released into the extracellular space and are designated as exosomes [1][3]. Evidence suggests that this process involves the endosomal sorting complex required for transport (ESCRT) function, which is made up of four ESCRT proteins that aid in membrane deformation and cargo selection and sorting [1][4]. But there are also ESCRT-independent methods of ILV formation and cargo selection such as via the ceramide-dependent mechanism or through tetraspanin proteins [5][6]. The mechanisms that regulate ILV and MVB formation are not well understood, although it is thought to be triggered by growth factors and cell homeostasis i.e. each cell produces exosomes adapted to its needs and environment [1].
The molecular constituents of exosomes depend substantially on their cell of origin. Environmental conditions, epigenetic changes, mechanisms of biogenesis and developmental stage also determine the molecular composition of exosomes which ultimately regulate their biological role [1].
1.2) Exosomes and the tumor microenvironment
Figure 1: Dual role of exosomes in cancer progression
Source: A. Von Schulze and F. Deng (2020) [7]
Crosstalk between different cells within the tumor microenvironment (TME), which includes stromal and immune cells, is vital for tumor growth and survival [2][8]. Communication between cancer cells and their surrounding cells occurs via cell-cell interactions, paracrine mechanisms and release of extracellular vesicles such as exosomes [1]. Exosomes play a dual role in tumorigenesis; depending on the cell of origin, exosomes released in the TME can either promote or inhibit tumor growth. As Figure 1 depicts, exosomes derived from cancer cells carry and deliver cargos that promote tumor growth, angiogenesis and metastasis, however, exosomes derived from healthy immune cells such as dendritic cells (DCs) and T cells can carry cargos that can inhibit tumor growth [7]. DCs release exosomes that can activate T cells, which in turn release exosomes that inhibit cancer cell growth, and then encourage NK cells to attack tumor cells. Therefore, exosomes involved in the TME which have an anti-tumoral role could potentially be used as drug candidates in their own right. Cancer cell derived exosomes which aid tumor progression could serve as drug carriers, these can be loaded with anti-cancer drugs and since they naturally target cancer cells, they may serve as an effective delivery system.
1.3) Naïve exosomes as therapeutics
Exosomes can have regenerative and protective effects, providing therapeutic benefit without being engineered i.e., naïve exosomes. As previously mentioned, exosomes released from immune cells in the TME such as DCs or T cells possess anti-tumor properties. Studies also demonstrate that mesenchymal stem cell (MSC)-derived exosomes are involved in the anti-inflammatory and regenerative effects of MSCs [9]–[12]. The therapeutic effect of MSCs appears to take place via paracrine function. By secreting cytokines, chemical molecules, and growth factors, MSCs can regulate the microenvironment and repair damaged tissue, and there is now evidence that demonstrates how exosomes play a key role in this [10]. MSCs are the most widely used stem cell in the clinic due to their regenerative potential, however, preclinical studies often fail efficacy expectations [10]. Since MSC-derived exosomes carry and can deliver miRNAs and proteins such as interleukins and growth factors which are able to inhibit expression of pro-inflammatory cytokines, they could become a potentially safer alternative to stem cell therapy with a lower manufacturing cost [9][13]. Moreover, exosomes are generally less immunogenic than cells, likely because they have fewer transmembrane proteins, such as MHC complexes, on their surface. Exosomes can also be frozen and stored more easily and for longer periods than cells, making them a more attractive alternative to cell therapies in terms of storage and logistics [13]. Evidence suggests that MSC-derived exosomes could be used to treat several diseases such as cardiovascular, neurodegenerative, and cerebrovascular diseases as well as wound repair [14][15][16][17]. Whether they have the potential to treat cancer is still unknown, since evidence is contradictory on whether they promote or inhibit tumor growth [18]. The application of naïve exosomes as therapeutics is a ‘hit-and-miss’ approach and unfortunately most studies up to now have failed to demonstrate consistent efficacy.
1.4) Exosomes as drug delivery systems
The potential of exosomes is not solely based on their natural therapeutic effects; current interest focuses on them as ideal vectors to carry and deliver drug cargos. There are several advantages that these microvesicles could bring to the drug delivery field. Firstly, exosomes are naturally occurring and have low immunogenicity, they have significantly less toxicity than lipid nanoparticles (LNPs) and have better in-vivo stability and distribution [13]. Exosomes are also thought to have a better delivery efficiency than LNPs as they can cross tissue and cellular barriers, with some exosomes also having tropism for particular cells or tissues which could limit off-target effects [19]. They can also be engineered to display specific ligands on their surface to target cells or tissue and can be loaded with a variety of different drug cargos which can either be presented on the surface or carried within the exosome as a way of protection from degradation whilst in circulation. Despite advances in the field, many challenges with exosome therapies remain, such as the need to optimize efficient exosome payload uptake, tropism and biodistribution, avoidance of lysosomal destruction and large-scale manufacturing.
2) Key Players in Oncology
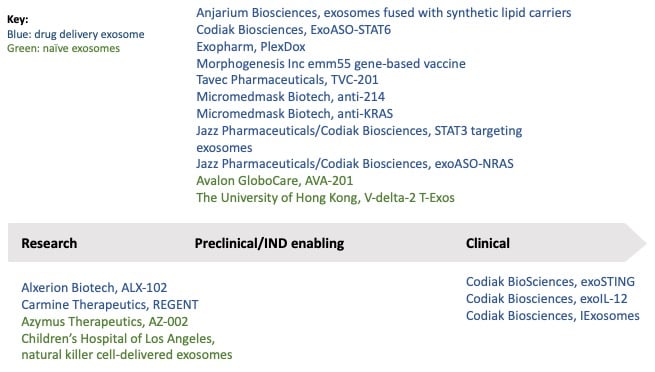
Figure 2: Oncology exosome projects by phase of development. The majority are in the preclinical phase and only four of these are naïve exosome therapies.
Exosome therapies have yet to reach the market, and only one company has managed to reach clinical development in the oncology space: Codiak BioSciences with exoSTING and exoIL-12. Around 13 companies and institutions are currently developing exosome therapies for oncology indications; these are outlined in Figure 2 [20]. The majority of these are in preclinical stage, and only four are naïve exosomes.
Although the field is relatively undeveloped, it is diverse with over 14 different types of cancers being targeted and a range of different payloads being explored (Table 1). The most popular payloads seem to be RNA based, although several companies have not disclosed their choice of payload or target oncology indication(s). Solid tumors in general seem to be the most targeted indication category, likely because exosomes offer potential for targeted tumor tropism and uptake.
Table 1: Competitive landscape overview by cancer type and payload. X represents single cancer indication; O represents various cancer indications.
2.1) Drug delivery exosomes
2.1.1) Codiak BioSciences: Current leader in the oncology exosome field
Codiak BioSciences is currently the leading company developing exosomes for drug delivery in oncology. Its proprietary platform technology, engEx™ was developed for designing, engineering, and manufacturing exosome therapeutics. Codiak reports that it can generate large volumes of exosomes with 2,000L fed-batch cell cultures and 500L perfusion bioreactors in suspension; so far this is the largest scale that has been reported. The platform allows Codiak to engineer exosomes to direct tropism and to load them with a wide range of therapeutics such as small molecules and nucleic acids, however, it is not clear whether delivery of large gene payloads has yet been achieved.
Codiak’s most advanced program, exoSTING, is in phase I/II clinical development for advanced solid tumors including anaplastic thyroid cancer, metastatic breast cancer, metastatic head and neck cancer, skin cancer and squamous cell carcinoma. The exosomes are loaded with a cyclic dinucleotide (CDN) small molecule ‘stimulator of interferon genes’ (STING) agonist. The STING pathway has been shown to incite an anti-tumoral immune response, but development of free STING therapeutics has been limited due to non-selective cell delivery resulting in off-target toxicity as well as leakage into the circulation causing dose-related toxicity. In vitro and in vivo data showed that exoSTING was preferentially taken up by differentiated antigen presenting cells (APCs) and elicited an anti-tumor immune response with greater potency than a free STING agonist [21], providing a safer and more potent alternative to free STING agonists [22].
Codiak’s other clinical stage program, exoIL-12 is in phase I for early-stage cutaneous T cell lymphoma with plans to expand into other IL-12 responsive solid tumors. Exosomes are loaded with surface IL-12 which promotes local anti-tumor activity; historically, systemic IL-12 leakage has caused toxicity and limited its therapeutic potential [23]. In preclinical studies, exoIL-12 delivered low dose IL-12 to achieve local and systemic tumor shrinkage, and demonstrated dose dependent injection-site retention with no measurable systemic exposure of IL-12 [24].
Codiak has three other programs in preclinical development: one being exoASO-STAT6 and two others in partnership with Jazz Pharmaceuticals, exoASO-NRAS and STAT3-targeting exosomes. ExoASO-STAT6 is being investigated for myeloid rich cancers and consists of engineered exosomes loaded with a linker-conjugated antisense oligonucleotide (ASO) targeting the STAT6 transcription factor, to reprogram tumor-associated M2 phenotype macrophages into M1, the anti-tumor phenotype [25]. STAT3-targeting exosomes inhibit STAT3 on tumor cells in both hematological malignancies and solid tumors [20]. Finally, exoASO-NRAS consists of exosomes loaded with an ASO that targets and decreases NRAS, a commonly mutated oncogene in cancer. It induces targeted anti-tumor activity and is being investigated in both liquid and solid tumors [20].
2.1.2) Exosomes as vectors for RNA therapies
RNA-based therapies hold great potential in the oncology field, offering an opportunity to hit previously undruggable targets. However, RNA drugs come with challenges, particularly for intracellular delivery given their high electric charge and larger size compared to small molecules [26]. Moreover, fine-tuning tropism for the right cells/tissues has proved difficult since upon systemic delivery, naïve forms of RNA tend to accumulate in the liver and kidneys and less so in other tissues and tumors [27]. Exosomes could provide a solution by carrying RNA payloads within the lumen whilst displaying sophisticated ligand-receptor systems on their surface to target specific tumors and then intracellularly releasing the RNA payloads. Several companies are investigating the potential of exosomes as RNA therapy carriers such as: Tavec Pharmaceuticals, Carmine Therapeutics, Anjarium and Micromedmark Biotech.
ASOs and siRNA therapies are some of the most advanced RNA therapeutic modalities; several companies are investigating exosomes as vectors for delivery of these therapies. Carmine Therapeutics uses red blood cell derived extracellular vesicles (RBCEVs) which are loaded with nucleic acids such as 125b-ASO and Cas9 mRNA. Although still in early development stage, there is promising data for 125b-ASO loaded RBCEVs in leukemia (MOLM13) and breast cancer (CA1a) cell lines, as well as inhibition of breast tumor growth after intratumoral injection of 125b-ASO-loaded RBCEVs into luciferase-labelled CA1a tumors in vivo [20][28]. Micromedmark Biotech is developing siRNA and RNAi exosome-based therapies, both of which are in preclinical stages of development. One is an anti-KRAS therapy consisting of exosomes loaded with siRNA that silences the KRAS oncogene, it is being investigated for treatment of lung and pancreatic tumors. The second product is an anti-214 delivered by exosomes, comprising an RNAi that silences tumor-secreted miR-214 (an oncogenic miRNA) [20].
Tavec Pharmaceuticals is developing TVC-201, a miRNA exosome therapy to treat cholangiocarcinoma (CCA), consisting of exosomes loaded with miR-195 which is downregulated in CCA. In one study, overexpression of miR-195 inhibited growth of four different CCA cell lines [29]. In in vivo models, miRNA-195-loaded exosomes inhibited cancer growth and prolonged lifespan [20].
2.1.3) Exosomes carrying bacterial genes
Another approach is to load exosomes with bacterial genes to target inaccessible tumors, whilst avoiding immunogenicity concerns. For example, gene therapies are commonly delivered via viral vectors such as AAVs. However, repeated dosing with AAV often results in an immune response as the body recognizes the virus as foreign and deploys a neutralizing antibody attack, limiting the dose a patient can receive [30]. Morphogenesis Inc. has partnered with the Karolinska Institute to load its injectable targeted exosomes with the bacterial gene encoding emm55 antigen for the potential treatment of multiple myeloma and other cancers. This project is early stage but in vivo studies demonstrated anti-tumor efficacy in a murine melanoma model [31][32]. This approach may be beneficial in overcoming typical immunogenicity and repeat dossing issues that come with standard gene therapy methods.
2.1.4) Exosomes as carriers for chemotherapeutic agents
Whilst most exosome therapies in development are based on more innovative approaches such as incorporating RNA therapeutics or gene therapies, some companies are utilizing exosomes to improve established oncology chemotherapeutics in two contexts. Firstly, chemotherapy often induces dose-limiting toxicity which has a major impact on patient quality of life and can therefore limit the potential benefits of the treatment. Secondly, chemotherapy resistance or disease recurrence occurs due to the inability of chemotherapeutic agents to eliminate cancer stem cells (CSCs), a small population of stem-like cells which play a key role in tumor development, progression and metastasis [33]. A study published in 2019 reported that tumor cell-derived exosomes loaded with doxorubicin, a widely used chemotherapeutic agent, resulted in a robust cytotoxic response against bulk cancer cells and CSCs as well as enhanced drug accumulation in the tumor (including in CSCs), extravasation from blood vessels and deep penetration into tumor parenchyma, improving the efficacy of doxorubicin and reducing its toxicity [34].
Exopharm is one of the leading companies in this field, with three exosome products in development and a well characterized platform for exosome isolation, purification, and engineering. PlexoDOX is in preclinical development for cancer; platelet derived exosomes are loaded with doxorubicin for more targeted delivery and enhanced efficacy of the chemotherapeutic agent against tumor cells [35]. In vitro data showed that PlexoDOX kills significantly more lung cancer cells than doxorubicin alone, at similar doses. Although this data is encouraging, in vivo studies are needed to further assess the potential of this product [35].
Alxerion Biotech is also developing a chemotherapeutic loaded exosome ALX-102, which is in its early stages of development for lung cancer. The product consists of cellular vesicles derived from a patient’s own non-small cell lung cancer (NSCLC) cells which are inactivated, purified, and loaded with methotrexate (MTX), a widely used chemotherapeutic agent. Studies showed that ALX-102 significantly inhibited NSCLC proliferation and was 150-fold more potent than MTX alone. By using the patient’s own exosomes, Alxerion expects to significantly reduce toxicity and side effects; however, no recent updates have been reported on this program [20].
2.2) Naïve exosomes
Some organizations are developing exosome therapeutics from naïve exosomes including Azymus Therapeutics, Avalon GloboCare, the University of Hong Kong and the Children’s Hospital of Los Angeles.
Avalon GloboCare is genetically modifying MSCs for high expression of miR-185 as a “bio-factory” to mass produce and isolate miR-185-enriched exosomes (AVA-201) for the potential topical treatment of oral cancer. The program was expected to enter the clinic in early 2020 after promising preclinical data demonstrated that AVA-201 inhibited oral potentially malignant disorder (OPMD) progression, suppressed inflammation, and activated the apoptotic pathway via direct targeting of AKT [20][36], however, no progress has been reported since.
Azymus Therapeutics uses the AZTM platform technology to produce primary human cell (PHC) derived exosomes carrying different payloads depending on the target disease. Instead of loading isolated exosomes with different payloads, it generates exosomes, from certain cell types, already carrying the desired payloads by altering the mediator formulation including specific cytokines and growth factors. The company’s oncology drug discovery program, AZ-002, disrupts the communications between CSCs and the TME in chemo-resistant cancer models. Early in vitro data showed promising effects in resensitization of certain drug-resistant tumors, but it is unknown how AZ-002 produces these effects [20][37].
The role of natural killer (NK) cells in immune activation against infected or abnormal cells makes them important as cancer immunotherapies. The Children’s Hospital of Los Angeles is developing NK-derived exosomes that stimulate anti-tumor activities. The NK cells are exposed to neuroblastoma cells, resulting in release of exosomes with a heightened cytotoxicity effect on neuroblastoma cells [38]. This project is still early stage and the hospital is looking to out-license the program [20].
Other immune cell derived exosomes are under investigation by the University of Hong Kong; for example V-delta-2-T cell derived exosomes for the potential treatment of Epstein-Barr virus (EBV)-associated tumors. To date, their use in the clinic has been limited by challenges in expanding autologous gamma delta T cells. A study conducted by the University demonstrated that allogenic V-delta-2-T cell derived exosomes had a greater therapeutic effect than autologous V-delta-2-T cell derived exosomes, which could potentially overcome the current production barriers [39].
3) Technical Challenges
Exosomes and their potential use as therapeutics is a relatively novel concept and several technical challenges remain to be resolved in the field. Currently, producing exosomes at a large scale is technically challenging and requires several steps. The choice of payload must be compatible, and sophisticated loading techniques must be developed to achieve efficient loading. One of the biggest advantages of using exosomes is the potential to target specific cells or tissues, but this of course means their tropism and biodistribution tendencies must be well characterized. Finally, it is not clear how well exosomes can escape lysosomal destruction; this would diminish their therapeutic effect.
3.1) Isolation and purification of exosomes
Until recently, exosomes were solely used for research purposes, not requiring mass production. As exosome programs begin to move into the clinic as therapeutics, concerns over scalability arise, particularly around efficient isolation and purification.
Firstly, it is important to select an appropriate source of exosomes e.g., cell lines that have established manufacturing and scalable processes, or a source of primary cells that are widely available to achieve a good yield of exosomes. For example, MSCs are widely used and their production method is well established; red blood cells (RBCs) are readily available and large quantities of exosomes can be obtained from a single unit of blood, removing the need for cell culturing. Large-scale amounts of extracellular vesicles can be purified from RBCs after treatment with calcium ionophore, for example, Carmine Therapeutics reports a production scale of 1013-1014 of exosomes purified from RBCs isolated from 200ml of blood [28].
Isolation and purification of exosomes must also be optimized for yield. Common techniques such as ultracentrifugation is used but this still has major scalability limitations. Other options include size-based separation techniques such as ultrafiltration or size exclusion chromatography, but these have additional issues such as low purity, damage by shear stress, low sample capacity and dilution of samples. Another purification technique, immunoaffinity chromatography (IAC), relies upon identifying molecules that are externally located on the exosome membrane. However, exosomes obtained by IAC have relatively harsh storage conditions, resulting in interfering proteins. So whilst IAC is well-suited to laboratory use, it is not yet optimized for large scale therapeutic exosome production [9][40][41].
Some companies are developing proprietary platforms with sophisticated isolation and purification techniques. One example is Exopharm with its LEAP Ligand technology which uses affinity chromatography to capture exosomes by binding to the actual exosome membrane rather than specific surface molecules. Exopharm claims to have industrial-sized columns that can produce millions of doses [42]. Codiak’s platform uses advanced chromatography and filtration technology for exosome purification, claiming a single run can produce an equivalent volume of purified exosomes that would traditionally require >2,000 centrifuge runs [43].
3.2) Loading of payloads
Once exosomes have been produced, isolated and purified the next step is to load them with the required drug cargos. The three major loading techniques used are incubation, electroporation and sonication. Incubation is the most widely used method [44], although the encapsulating rate and efficiency is relatively low with this approach [45]. Electroporation can often be complemented with incubation to enhance loading efficiency by applying an extra electrical field that enhances exosome permeability [46]. However, electroporation can lead to RNA precipitation or exosome aggregation, limiting loading efficiency in some cases [45]. A study investigating loading efficiency of paclitaxel with each of these three methods showed that sonication offers the highest loading efficiency (29% compared to 1.5% and 5.3% for incubation and electroporation, respectively) [47]. However, sonication weakens of the exosome membrane, affecting the surface protein structure and causing exosome aggregation [44][46].
Another popular method is transfection of the parental cells, which theoretically offers a higher loading efficiency and molecular stability than other methods, but transfection agents have toxicity and safety concerns which may alter gene expression in exosome producing cells, thereby affecting nucleic acid drug cargos and thus the biological activity of the exosomes. Other less commonly used strategies such as freeze-thaw cycles, thermal shock, pH gradient, saponin-assisted loading, extrusion, and hypotonic dialysis could be used, but their cons tend to outweigh their pros [45].
More sophisticated strategies are being developed to increase loading efficiency and maintain exosome fidelity. An interesting example of this is Anjarium Bioscience and its “hybridosome” technology which leverages the loading capacity of synthetic vesicles to efficiently load exosomes with RNA cargos. The synthetic vesicles are designed to carry these cargos which are released within the exosome upon fusion [48].
Ilias Biologics’ sophisticated protein loading technology works via an optically reversible protein-protein interaction, in which two light-sensing proteins from the plant Arabidopsis thaliana, CRY2 and CIBN, reversibly bind to each other when exposed to blue light. Ilias leverages this naturally occurring protein interaction by engineering exosome producing cells to express two sets of protein complexes: CRY2-conjugated cargo protein and CIBN-conjugated exosome membrane-associated protein. These cells are cultured under blue light, resulting in the CRY2-CIBN interaction, dragging the protein attached to CRY2 into the exosome [49]. Ilias Biologics is in early discovery stages of developing an anti-tumor exosome therapy of intracellular antibodies; as yet there is no information available regarding this program.
3.3) Tissue/cell tropism and Biodistribution
One of the biggest unmet needs in cancer treatment is the lack of cancer cell selectivity with targeted therapies. Naturally, derived exosomes have specific surface protein profiles that allow targeting of certain cells/tissues. For example, MSC-derived exosomes or fibroblasts can target tumor cells via their surface proteins, allowing them to interact via cancer cell surface receptors and/or release intracellular payloads, such as miRNAs, to promote cancer progression and induce drug resistance [50]–[52]. These exosomes could therefore be engineered to carry anti-cancer drugs which can be delivered directly to tumor cells and internalized. The exosome surface could also be modified to include ligands that target certain cells or tissues.
Codiak employs methods for “compartmental” delivery (e.g., intratumoral, intrathecal) of exosomes to ensure enough drug gets to the desired target while limiting potential off-target side effects. The company combines this strategy with engineering the surface proteome of exosomes for selective targeting [53]. Other companies have developed platforms for surface modification of exosomes, including Exopharm’s EVPS™ platform or Anjarium’s ‘targeting technology’ which decorates hybridosomes with PEG, antibody fragments and peptides [54][55].
Other methods include careful cell line sourcing e.g., Tavec uses a human liver stellate cell line (LX2) to develop its CCA therapy. LX2-derived exosomes have tropism for CCA cells, so Tavec is essentially exploiting the cancer cell’s natural pre-disposition for accumulating large quantities of certain exosomes [29]. Another example is work being done at The Children’s Hospital of Los Angeles where exposing NK cells to neuroblastoma cells results in release of exosomes that are able to activate other NK cells to exclusively attack neuroblastoma cells [38].
3.4) Lysosomal destruction
One of the threats to exosomes, once they are internalized by target cells, is lysosomal destruction. A study visualizing the internalization and colocalization of exosomes showed that once in the target cell, large portions of exosomes are transported to lysosomes for degradation [56]. So far, little to no data is available on how or if therapeutic exosomes can escape lysosomal degradation. Further studies to characterize exosome fate are needed.
4) Conclusions and future outlook
In 2020, there were approximately 19.3 million new cancer cases worldwide and almost 10 million deaths, ranking cancer as the leading cause of death globally [57]. There is an urgent need for novel effective targeted therapies that can potently and selectively target cancer cells, without eliciting side effects. Exosomes clearly have the potential to revolutionize the cancer drug delivery landscape due to their low immunogenicity, ability to target specific cells and carry complex cargos. Naïve exosome therapeutics could serve as alternatives to cell therapy which still suffers from manufacturing and logistic challenges that have to date limited its use in the clinic.
Bibliography
[1] Y. Zhang, Y. Liu, H. Liu, and W. H. Tang, “Exosomes: biogenesis, biologic function and clinical potential,” Cell Biosci. 2019 91, vol. 9, no. 1, pp. 1–18, Feb. 2019, doi: 10.1186/S13578-019-0282-2.
[2] J. Dai et al., “Exosomes: key players in cancer and potential therapeutic strategy,” Signal Transduction and Targeted Therapy, vol. 5, no. 1. 2020, doi: 10.1038/s41392-020-00261-0.
[3] I. A and M. E, “Exosomes: Fundamental Biology and Roles in Cardiovascular Physiology,” Annu. Rev. Physiol., vol. 78, pp. 67–83, Feb. 2016, doi: 10.1146/ANNUREV-PHYSIOL-021115-104929.
[4] W. M. Henne, N. J. Buchkovich, and S. D. Emr, “The ESCRT Pathway,” Dev. Cell, vol. 21, no. 1, pp. 77–91, Jul. 2011, doi: 10.1016/J.DEVCEL.2011.05.015.
[5] B. M. Castro, M. Prieto, and L. C. Silva, “Ceramide: A simple sphingolipid with unique biophysical properties,” Prog. Lipid Res., vol. 54, no. 1, pp. 53–67, Apr. 2014, doi: 10.1016/J.PLIPRES.2014.01.004.
[6] D. Perez-Hernandez et al., “The Intracellular Interactome of Tetraspanin-enriched Microdomains Reveals Their Function as Sorting Machineries toward Exosomes *,” J. Biol. Chem., vol. 288, no. 17, pp. 11649–11661, Apr. 2013, doi: 10.1074/JBC.M112.445304.
[7] A. Von Schulze and F. Deng, “A review on exosome-based cancer therapy,” J. Cancer Metastasis Treat., vol. 2020, 2020, doi: 10.20517/2394-4722.2020.79.
[8] C. Roma-Rodrigues, A. R. Fernandes, and P. V. Baptista, “Exosome in Tumour Microenvironment: Overview of the Crosstalk between Normal and Cancer Cells,” Biomed Res. Int., vol. 2014, 2014, doi: 10.1155/2014/179486.
[9] T. Yamashita, Y. Takahashi, and Y. Takakura, “Possibility of Exosome-Based Therapeutics and Challenges in Production of Exosomes Eligible for Therapeutic Application,” Biol. Pharm. Bull., vol. 41, no. 6, pp. 835–842, Jun. 2018, doi: 10.1248/BPB.B18-00133.
[10] W. Wei et al., “Mesenchymal Stem Cell–Derived Exosomes: A Promising Biological Tool in Nanomedicine,” Front. Pharmacol., vol. 11, p. 1954, Jan. 2021, doi: 10.3389/FPHAR.2020.590470.
[11] X. Wang et al., “Exosomal miR-223 Contributes to Mesenchymal Stem Cell-Elicited Cardioprotection in Polymicrobial Sepsis,” Sci. Rep., vol. 5, Sep. 2015, doi: 10.1038/SREP13721.
[12] “Exosome secreted by MSC reduces myocardial ischemia/reperfusion injury | Elsevier Enhanced Reader.” https://reader.elsevier.com/reader/sd/pii/S187350610900141X?token=08CFD88F66D16DD98140198452DDAB9D9432EF883EEE80AF589A1F742D349D263BEC23980768845A7C0E5E235F5B825B&originRegion=eu-west-1&originCreation=20210902084108 (accessed Sep. 02, 2021).
[13] T. Krol, J. West, J. Hayden, and M. He, “Exosomes - The Good, Bad, Ugly and Current State,” American Pharmaceutical Review, 2021. https://www.americanpharmaceuticalreview.com/Featured-Articles/575432-Exosomes-The-Good-Bad-Ugly-and-Current-State/.
[14] C. GH et al., “Exosomes derived from hypoxia-preconditioned mesenchymal stromal cells ameliorate cognitive decline by rescuing synaptic dysfunction and regulating inflammatory responses in APP/PS1 mice,” FASEB J., vol. 32, no. 2, pp. 654–668, Feb. 2018, doi: 10.1096/FJ.201700600R.
[15] R. M et al., “Stem Cell-Derived Exosomes as Nanotherapeutics for Autoimmune and Neurodegenerative Disorders,” ACS Nano, vol. 13, no. 6, pp. 6670–6688, Jun. 2019, doi: 10.1021/ACSNANO.9B01004.
[16] M. GJ et al., “Application of Mesenchymal Stem Cell-Derived Extracellular Vesicles for Stroke: Biodistribution and MicroRNA Study,” Transl. Stroke Res., vol. 10, no. 5, pp. 509–521, Oct. 2019, doi: 10.1007/S12975-018-0668-1.
[17] F. Rao et al., “Exosomes from human gingiva-derived mesenchymal stem cells combined with biodegradable chitin conduits promote rat sciatic nerve regeneration,” Stem Cells Int., vol. 2019, 2019, doi: 10.1155/2019/2546367.
[18] A. Hmadcha, A. Martin-Montalvo, B. R. Gauthier, B. Soria, and V. Capilla-Gonzalez, “Therapeutic Potential of Mesenchymal Stem Cells for Cancer Therapy,” Front. Bioeng. Biotechnol., vol. 0, p. 43, Feb. 2020, doi: 10.3389/FBIOE.2020.00043.
[19] D. E. Murphy et al., “Extracellular vesicle-based therapeutics: natural versus engineered targeting and trafficking,” Exp. Mol. Med. 2019 513, vol. 51, no. 3, pp. 1–12, Mar. 2019, doi: 10.1038/s12276-019-0223-5.
[20] “Home Page.” https://www.cortellis.com/intelligence/home.do (accessed Sep. 06, 2021).
[21] S. Chul Jang et al., “Selective Activation of Antigen Presenting Cells by exoSTING Enhances Tumor Antigen-Specific Immune Response.”
[22] “exoSTINGTM • Codiak Biosciences.” https://www.codiakbio.com/pipeline-programs/exosting (accessed Sep. 07, 2021).
[23] “exoIL-12TM • Codiak Biosciences.” https://www.codiakbio.com/pipeline-programs/exoil-12 (accessed Sep. 07, 2021).
[24] S. Chul Jang, “Exosomes are natural, cell-derived vesicles that can act as a non-immunogenic delivery system for multiple therapeutic payloads IL-1-Stimulated Human NK Cells IL-12 Concentration (ng/ml) IFN (RLU) Exosome Surface Display of IL-12 Results in Tumor-Retained Pharmacology with Superior Potency and Limited Systemic Exposure Compared to Recombinant IL-12.”
[25] “exoASOTM-STAT6 • Codiak Biosciences.” https://www.codiakbio.com/pipeline-programs/exoaso-stat6 (accessed Sep. 09, 2021).
[26] Y.-K. Kim, “RNA Therapy: Current Status and Future Potential,” Chonnam Med. J., vol. 56, no. 2, p. 87, 2020, doi: 10.4068/CMJ.2020.56.2.87.
[27] A. R. MacLeod and S. T. Crooke, “RNA Therapeutics in Oncology: Advances, Challenges, and Future Directions,” J. Clin. Pharmacol., vol. 57, no. S10, pp. S43–S59, 2017, doi: 10.1002/JCPH.957.
[28] W. M. Usman et al., “Efficient RNA drug delivery using red blood cell extracellular vesicles,” Nat. Commun. 2018 91, vol. 9, no. 1, pp. 1–15, Jun. 2018, doi: 10.1038/s41467-018-04791-8.
[29] L. L et al., “Extracellular vesicles carry microRNA-195 to intrahepatic cholangiocarcinoma and improve survival in a rat model,” Hepatology, vol. 65, no. 2, pp. 501–514, Feb. 2017, doi: 10.1002/HEP.28735.
[30] M. Cully, “Exosome-based candidates move into the clinic,” Nat. Rev. Drug Discov., vol. 20, no. 1, pp. 6–7, Jan. 2021, doi: 10.1038/D41573-020-00220-Y.
[31] B. L. Bunch et al., “Anti-tumor efficacy of plasmid encoding emm55 in a murine melanoma model,” Cancer Immunol. Immunother. 2020 6912, vol. 69, no. 12, pp. 2465–2476, Jun. 2020, doi: 10.1007/S00262-020-02634-4.
[32] “Morphogenesis, Inc and the Cell and Gene Therapy Group at Karolinska Institutet Announce Collaborati - BioFlorida, Inc.” https://www.bioflorida.com/news/417721/Morphogenesis-Inc-and-the-Cell-and-Gene-Therapy-Group-at-Karolinska-Institutet-Announce-Collaborati.htm (accessed Sep. 14, 2021).
[33] V. JE and L. GJ, “Cancer stem cells in solid tumours: accumulating evidence and unresolved questions,” Nat. Rev. Cancer, vol. 8, no. 10, pp. 755–768, Oct. 2008, doi: 10.1038/NRC2499.
[34] T. Yong et al., “Tumor exosome-based nanoparticles are efficient drug carriers for chemotherapy,” Nat. Commun. 2019 101, vol. 10, no. 1, pp. 1–16, Aug. 2019, doi: 10.1038/s41467-019-11718-4.
[35] “EXOPHARM LIMITED ACN: 163 765 991 ASX:EX1 ASX ANNOUNCEMENT & MEDIA RELEASE,” Accessed: Sep. 13, 2021. [Online]. Available: http://exo.ph/ExoMails.
[36] W. L et al., “Delivery of mesenchymal stem cells-derived extracellular vesicles with enriched miR-185 inhibits progression of OPMD,” Artif. cells, nanomedicine, Biotechnol., vol. 47, no. 1, pp. 2481–2491, Dec. 2019, doi: 10.1080/21691401.2019.1623232.
[37] “AZTM Platform | Azymus Therapeutics.” https://azymustherapeutics.com/platform/ (accessed Sep. 17, 2021).
[38] A. Shoae-Hassani, A. A. Hamidieh, M. Behfar, R. Mohseni, S. A. Mortazavi-Tabatabaei, and S. Asgharzadeh, “NK Cell–derived Exosomes From NK Cells Previously Exposed to Neuroblastoma Cells Augment the Antitumor Activity of Cytokine-activated NK Cells,” J. Immunother., vol. 40, no. 7, p. 265, 2017, doi: 10.1097/CJI.0000000000000179.
[39] “HKUMed develops a novel therapeutic approach | EurekAlert!” https://www.eurekalert.org/news-releases/524819 (accessed Sep. 17, 2021).
[40] T. Liangsupree, E. Multia, and M. L. Riekkola, “Modern isolation and separation techniques for extracellular vesicles,” J. Chromatogr. A, vol. 1636, p. 461773, Jan. 2021, doi: 10.1016/J.CHROMA.2020.461773.
[41] Y. Zhang, J. Bi, J. Huang, Y. Tang, S. Du, and P. Li, “Exosome: A review of its classification, isolation techniques, storage, diagnostic and targeted therapy applications,” Int. J. Nanomedicine, vol. 15, pp. 6917–6934, 2020, doi: 10.2147/IJN.S264498.
[42] “Exopharm’s LEAP technology a breakthrough in exosome therapeutics - Stockhead.” https://stockhead.com.au/health/exopharms-leap-technology-a-breakthrough-in-exosome-therapeutics/ (accessed Sep. 17, 2021).
[43] “Manufacturing • Codiak Biosciences.” https://www.codiakbio.com/manufacturing (accessed Sep. 17, 2021).
[44] M. Yang and S. Y. Wu, “The Advances and Challenges in Utilizing Exosomes for Delivering Cancer Therapeutics,” Front. Pharmacol., vol. 0, no. JUL, p. 735, Jul. 2018, doi: 10.3389/FPHAR.2018.00735.
[45] X.-M. Xi, S.-J. Xia, and R. Lu, “Drug loading techniques for exosome-based drug delivery systems.,” Pharmazie, vol. 76, no. 2, pp. 61–67, Feb. 2021, doi: 10.1691/ph.2021.0128.
[46] S. Fu, Y. Wang, X. Xia, and J. C. Zheng, “Exosome engineering: Current progress in cargo loading and targeted delivery,” NanoImpact, vol. 20, p. 100261, Oct. 2020, doi: 10.1016/J.IMPACT.2020.100261.
[47] K. MS et al., “Development of exosome-encapsulated paclitaxel to overcome MDR in cancer cells,” Nanomedicine, vol. 12, no. 3, pp. 655–664, Apr. 2016, doi: 10.1016/J.NANO.2015.10.012.
[48] Y. Y. Jhan et al., “Engineered extracellular vesicles with synthetic lipids via membrane fusion to establish efficient gene delivery,” Int. J. Pharm., vol. 573, p. 118802, Jan. 2020, doi: 10.1016/J.IJPHARM.2019.118802.
[49] “ILIAS Biologics.” https://www.iliasbio.com/our/platform.php (accessed Sep. 18, 2021).
[50] H. Y et al., “Fibroblast-Derived Exosomes Contribute to Chemoresistance through Priming Cancer Stem Cells in Colorectal Cancer,” PLoS One, vol. 10, no. 5, May 2015, doi: 10.1371/JOURNAL.PONE.0125625.
[51] R. AM et al., “BM mesenchymal stromal cell-derived exosomes facilitate multiple myeloma progression,” J. Clin. Invest., vol. 123, no. 4, pp. 1542–1555, Apr. 2013, doi: 10.1172/JCI66517.
[52] W. J et al., “Bone marrow stromal cell-derived exosomes as communicators in drug resistance in multiple myeloma cells,” Blood, vol. 124, no. 4, pp. 555–566, Jul. 2014, doi: 10.1182/BLOOD-2014-03-562439.
[53] “Targeted Administration • Codiak Biosciences.” https://www.codiakbio.com/our-science/targeted-administration (accessed Sep. 20, 2021).
[54] “Products.” https://exopharm.com/products/ (accessed Sep. 20, 2021).
[55] J. DE Beer, “(54) (71) (72) (73) (21) (22) (86) (60) HYBRIDOSOMES, COMPOSITIONS COMPRISING THE SAME, PROCESSES FOR THER PRODUCTION AND USES THEREOF,” 2016.
[56] X. Li et al., “Challenges and opportunities in exosome research—Perspectives from biology, engineering, and cancer therapy,” APL Bioeng., vol. 3, no. 1, p. 11503, Mar. 2019, doi: 10.1063/1.5087122.
[57] H. Sung et al., “Global Cancer Statistics 2020: GLOBOCAN Estimates of Incidence and Mortality Worldwide for 36 Cancers in 185 Countries,” CA. Cancer J. Clin., vol. 71, no. 3, pp. 209–249, May 2021, doi: 10.3322/CAAC.21660.
Product development support
Alacrita's can support you through every step of the pharmaceutical product development process, leveraging our scientific, clinical and commercial expertise to help you maximize value at each stage. Please click a service below to learn more or contact us.
Product development services we provide: